4 Rocks and the Rock Cycle
Nahgeib Miller
Learning Objectives
After completing this chapter, you should be able to:
- Define what rock is.
- Describe and explain the formation of igneous rocks.
- Describe and explain the formation of sedimentary rocks.
- Describe and explain the formation of metamorphic rocks.
- Explain how the three classes of rock are interrelated through the rock cycle.
4.1 An Introduction to Rocks
Rocks are an amalgam of the minerals that crystallize from the “soup of potential,” precipitate from solution or that are transformed from other existing minerals by heat and pressure. As a child, during my rock-collecting adventures, I was fascinated by the array of colours in rocks and by the way some of those colours seemed to sparkle in the light of the Sun. The different colours represented individual minerals in the rock, and the “sparkle” represented the minerals with good cleavage. Intuitively, I was able to recognize that a rock was not just one thing but the many different parts that made it up. More specifically, rock is a naturally occurring aggregate of minerals.
The types of minerals present in a rock and their relative proportions and sizes can help tell the story of how that rock came to be. They can indicate what kind of environment the rock formed in, how quickly it crystallized, the depth at which it formed, how far the mineral travelled, and (for sedimentary rocks) how it was deposited, even the kinds of tectonic forces acting on it after it formed! The minerals tell the history of the rock, form part of the rock record, and are used to identify them.
Rocks are classified on the basis of texture and composition. Texture is a visual description of the size of the minerals in the rock, including the relative sizes of the individual minerals that make up the rock. Generally speaking, minerals that can be seen without the aid of magnification are considered large or “coarse,” and those that cannot be seen without magnification are considered small or “fine.” Composition speaks to the relative proportions of the different types of minerals in a rock (Figure 4.1). Typically, minerals are identified using their physical properties (as discussed in Chapter 3), with the proportions of minerals expressed as a percentage.
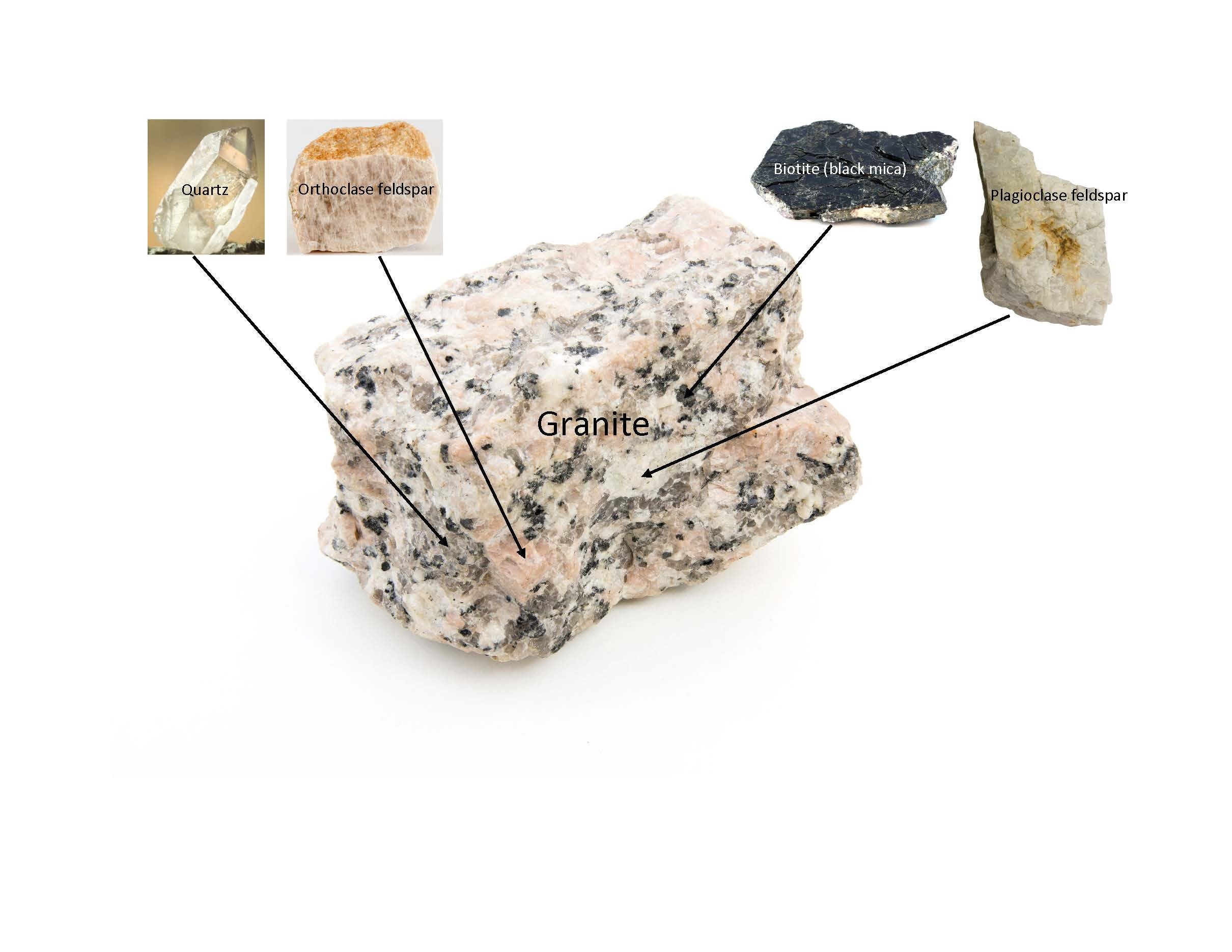
All rocks are classified into the three categories of igneous, sedimentary, and metamorphic rock. Igneous rocks are formed by the crystallization or solidification of magma or lava. Sedimentary rocks form when sediments derived from weathering are subsequently eroded, transported, deposited, buried, and lithified (turned into rock). Metamorphic rocks form from existing rocks in Earth’s crust that are exposed to high temperatures and pressures, which cause the minerals in the rocks to recrystallize in the solid state. That means that the crystal structure of the existing mineral changes without remelting.
4.2 Igneous Rocks
Igneous comes from the Latin word for “fire,” ignis. It is an apt name for these rocks because they form from the crystallization or solidification of molten rock (magma, lava). Igneous rocks are generally classified as either intrusive or extrusive.
Intrusive igneous rocks form from magma that cools and solidifies within Earth’s crust. As magma rises in the surrounding unmelted country rock in the crust and solidifies, it cools slowly, resulting in large mineral crystals that develop a coarse-grained texture. The relative sizes of the minerals in these rocks indicate a change or changes in the rate of cooling caused by magma rising from a deeper or shallower position in the crust. The presence of two distinct mineral sizes in intrusive igneous rock results in a specific type of coarse-grained texture called a porphyry. Porphyritic textures consist of a relatively large mineral, called a phenocryst, “floating” in a relatively small groundmass.
Extrusive igneous rocks form from magma that erupts at the surface as lava, which solidifies quickly, resulting in small mineral crystals that develop a fine-grained texture. Depending on the composition of the lava, the style of eruption can further dictate the size of the minerals and thus the type of rock. Lavas that have a low silica content (over about 60%) are less viscous and therefore tend to ooze out of fissures and volcanoes and solidify on the ground. Hawaiian-style eruptions are good examples of this. Higher-silica-content lavas (less than about 60%) will be more viscous, resisting flow. This resistance to flow can lead to a buildup of pressure, resulting in a violent eruption that throws the lava into the air. In the air, the lava separates into pieces of various sizes that solidify and fall to the ground. These rocks are called pyroclastic igneous rocks, with the largest pieces called volcanic bombs and the smallest pieces called volcanic ash (Video 4.1). Furthermore, the style of eruption is also controlled by the gas content in the magma, with higher amounts resulting in more violent eruptions.
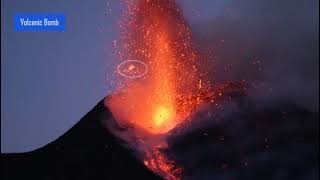
Formation of volcanic bombs and ash.
Click on the image to load the video.
Igneous Rock Classification Based on Mineral Composition and Texture
Although igneous rocks are generally classified as intrusive or extrusive, which is an indication of their texture (coarse or fine), they are further classified on the basis of their composition. Igneous rocks are classified into the two compositional categories of felsic and mafic.
Felsic igneous rocks are rocks that are rich in feldspar and silica (quartz) minerals. These minerals have a lower metallic content and therefore have lighter, less intense colours (clear, white, pink, and grey). This gives felsic igneous rocks an overall lighter colour than mafic igneous rocks (Figure 4.2).
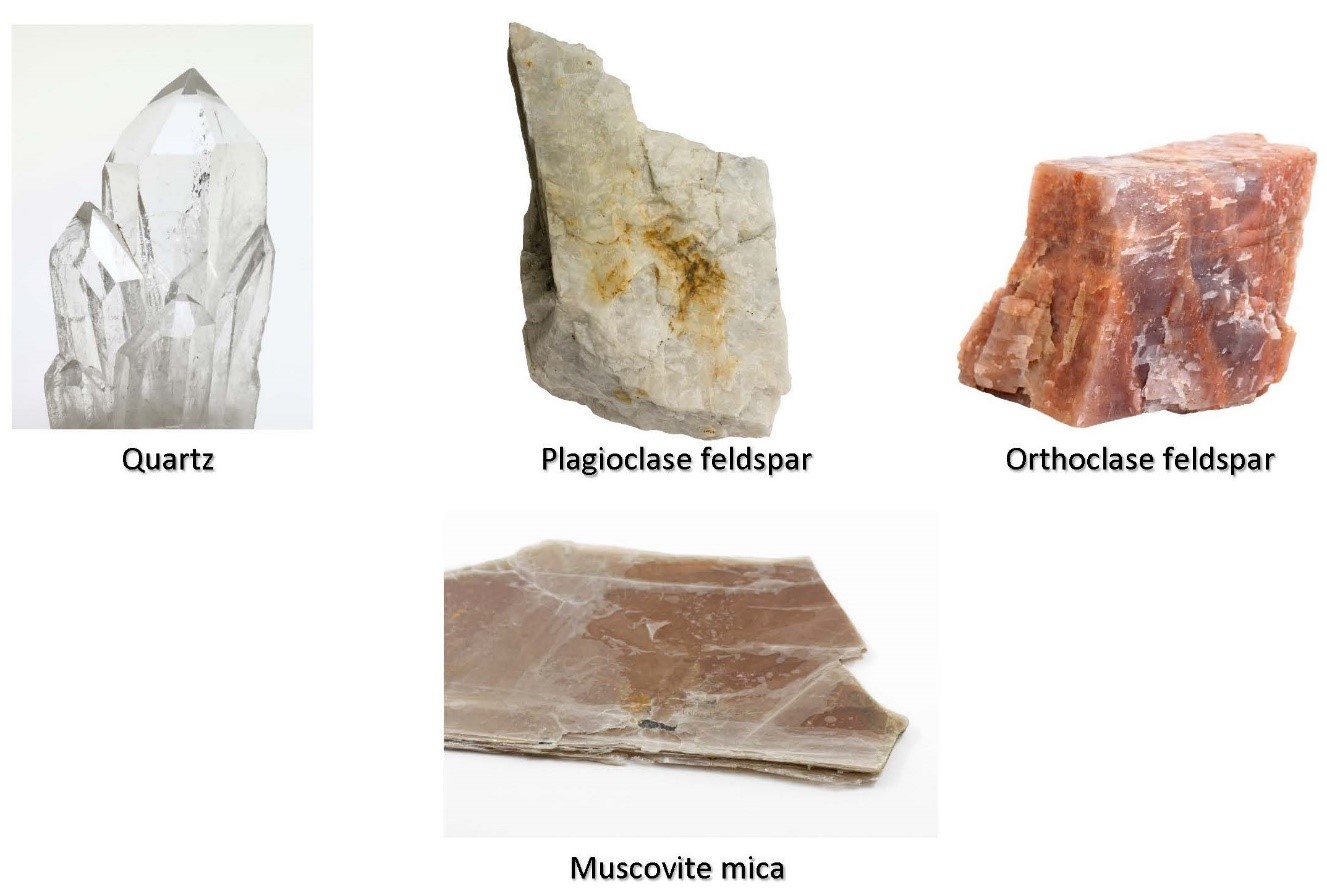
Quartz, orthoclase feldspar, plagioclase feldspar, muscovite mica (white mica)
Mafic igneous rocks are rocks that are rich in the metallic cations of magnesium and iron (ferric). Because metals tend to generate strong colours in minerals, mafic igneous rocks will have minerals that are darker than felsic igneous rocks (black, green, brown, red, dark grey; see Figures 4.3 and 4.5).
The relative proportion of dark and light-coloured minerals in a rock can be used to determine the overall mineral composition; therefore, igneous rocks can be classified into more specific compositional groups: felsic, intermediate, mafic, and ultramafic. The compositional grouping is then used in concert with the texture to derive a classification (Interactive Figure 4.1 and Figure 4.4).
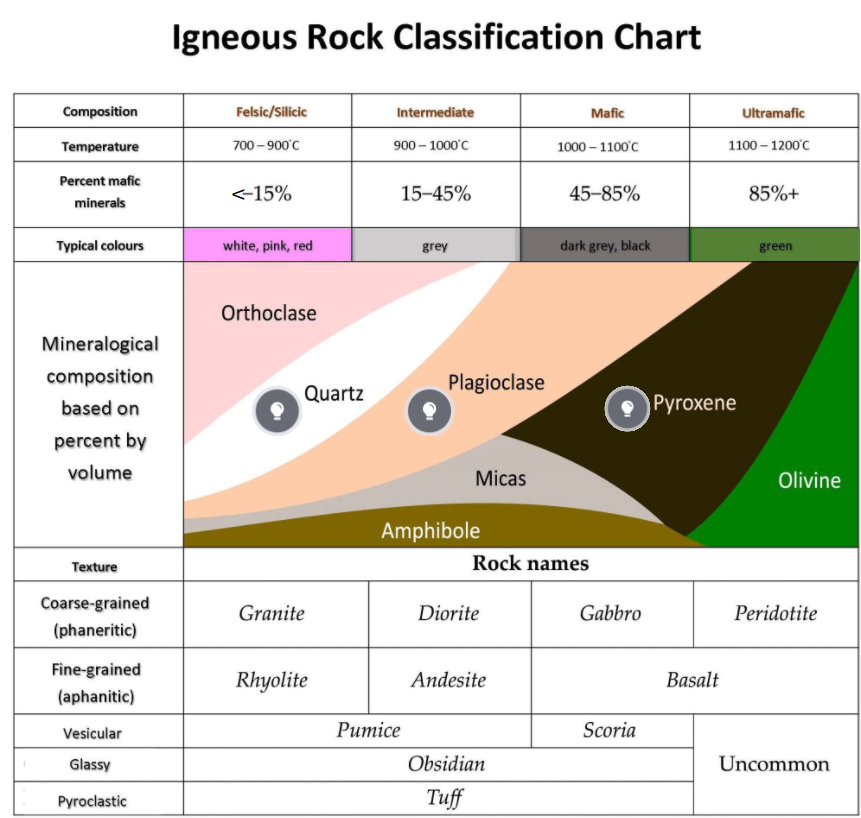
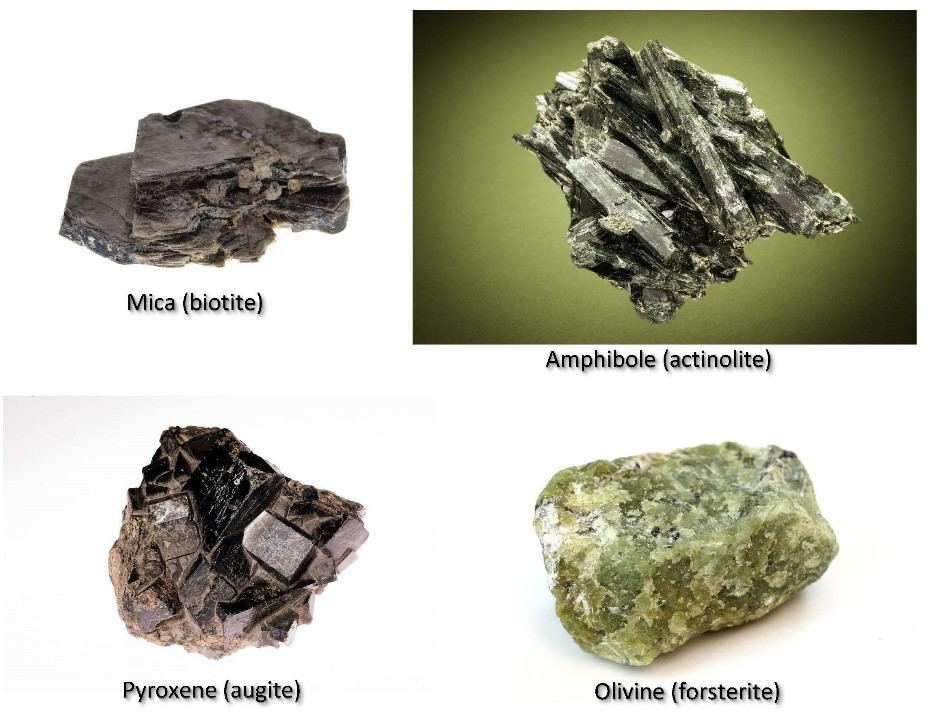
Biotite (black mica), amphibole group, pyroxene group, olivine group
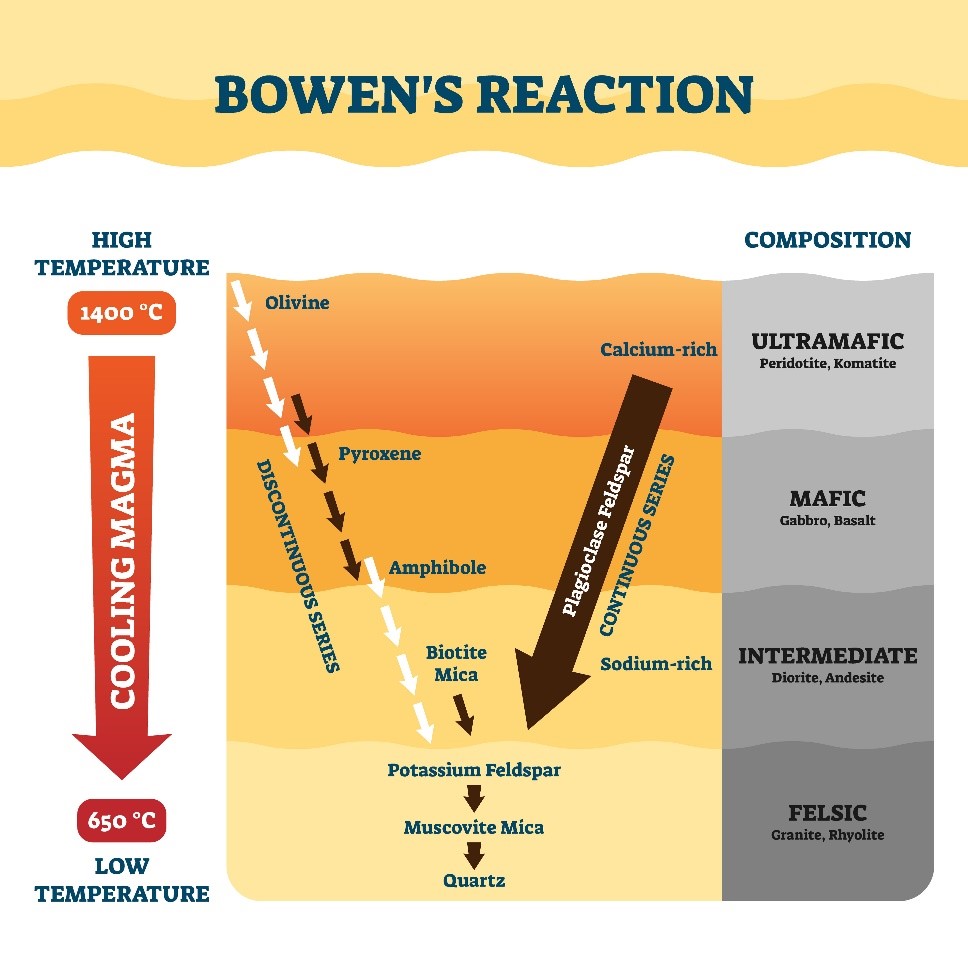
Note that the silica content and, therefore, the viscosity increase as temperature decreases. The density of the minerals also generally decreases in the direction of cooling due to the presence of larger cations
Igneous Geologic Structures
Extrusive igneous rocks are also called volcanic rocks because they tend to erupt onto the surface through volcanic activity. Intrusive igneous rocks are also known as plutonic rocks, and the structures that they make upon solidifying are called plutons. Plutons (Figure 4.5) are classified as either discordant or concordant intrusions.
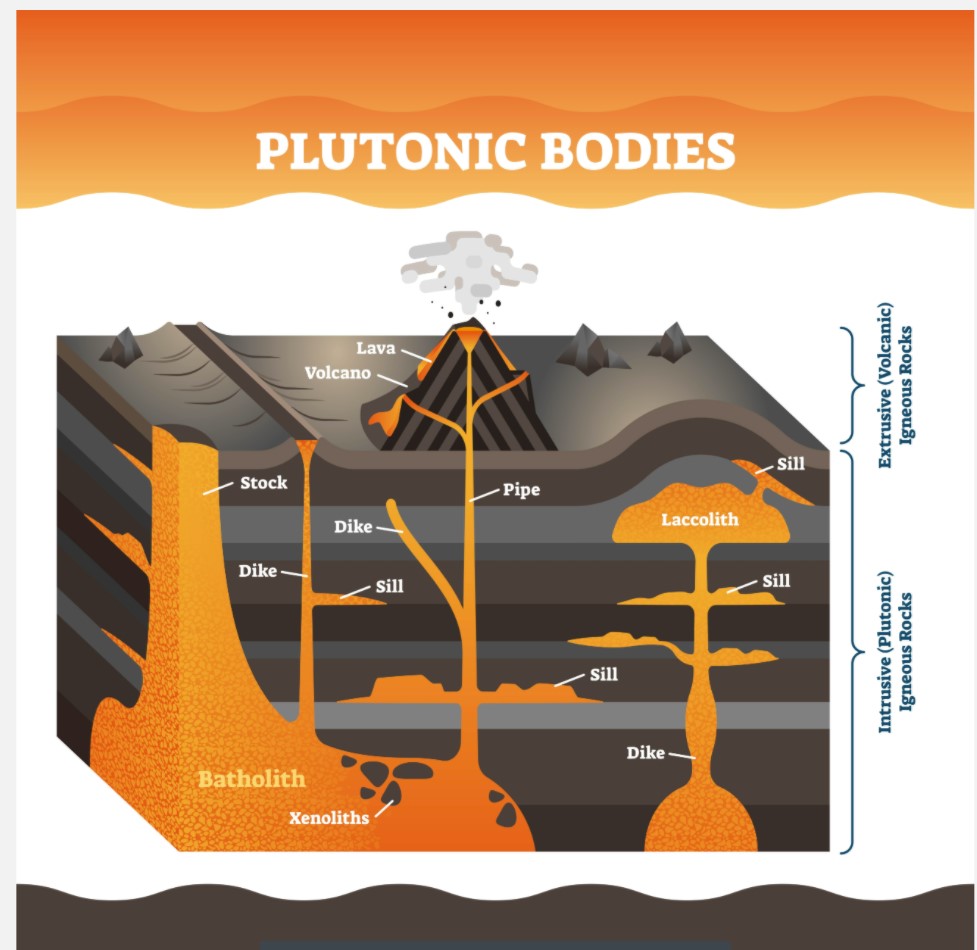
Discordant intrusions are magmatic intrusions that do not follow the prevailing geology of the country rock (bedding, faults, and joints). They are classified based on their size, with the largest being a batholith (hundreds of kilometres in width and thickness) and the smallest being a stock (kilometres in width and thickness).
Concordant intrusions are magmatic intrusions that follow cracks and natural zones of weakness in the surrounding country rock (bedding, faults, and joints). These are smaller than discordant intrusions. When the magma solidifies in a crack that cuts across the layers in the host rock, it is called a dike. When the magma solidifies in cracks that are irregular and localized, it is called a vein. Veins and dikes are essentially the same, but dikes follow more regular cross-cutting relationships with the host rock and will be wider and more continuous than veins. When magma solidifies along bedding directions (that is, parallel to the layers in the rock), it is called a sill.
4.3 Sedimentary Rocks
Sediments are created when existing rock is broken down at the surface of Earth through processes of weathering. Sediments can also be generated by the shells and skeletons of dead marine animals and from precipitation out of evaporating water. Sediments are transported under the influence of gravity through processes of erosion, fluvial transport (flowing water), or aeolian transport (wind), or ice transport (glaciers). When they come to rest, they form sedimentary rocks through processes of burial and diagenesis. These processes of weathering, erosion, transport, burial, and diagenesis leave an imprint on the sediments that can be used to determine the processes that acted on the sediment and their depositional environment. Now let us look at these processes in a bit more detail.
4.4 Sedimentary Rock Formation
An Introduction to Weathering
Weathering is the process that breaks down rock at the surface of Earth, resulting in smaller pieces called sediment. Note that sediment can range from as large as several metres in diameter to as small as 0.004 mm in diameter. Weathering can occur through physical or chemical forces (we will discuss this in more detail in Chapter 5). Physical weathering breaks down rock through mechanical forces like frost wedging, attrition, burrowing by organisms (including roots), while chemical weathering occurs due to chemical reactions of minerals in the rock with liquid water.
Minerals in rock weather at different rates because some minerals are more susceptible than others. In general, the minerals that form first out of the “soup of potential” are also the ones that weather and break down first. Therefore, olivine is more susceptible to weathering than quartz. Apart from the chemistry of a mineral, its internal crystal structure also plays a role in its susceptibility to weathering. We will discuss this further in Chapter 5. The differential rates of weathering cause the more resistant minerals to be “released” from the rock (that is, to be no longer bound by adjacent minerals), making them available for erosion and transport.
Erosion
Erosion is the process that removes the newly created sediments. Erosion can occur due to the effects of gravity or, more commonly, due to water flowing or wind passing over the surface of weathered rock.
Sediments are classified in three categories. Those derived from existing igneous rock are classified as siliciclastic (from Latin silex, meaning “hard stone,” “flint,” or “quartz,” and Greek klasis, meaning “breaking”). Sediments derived from chemical processes, such as precipitation due to the evaporation of water with dissolved minerals, are classified as chemical sediments. Sediments derived from the shells or skeletons of dead organisms are classified as biological sediments. These include carbonates from, for example, corals, and silica from microscopic organisms like diatoms (Figure 4.6).
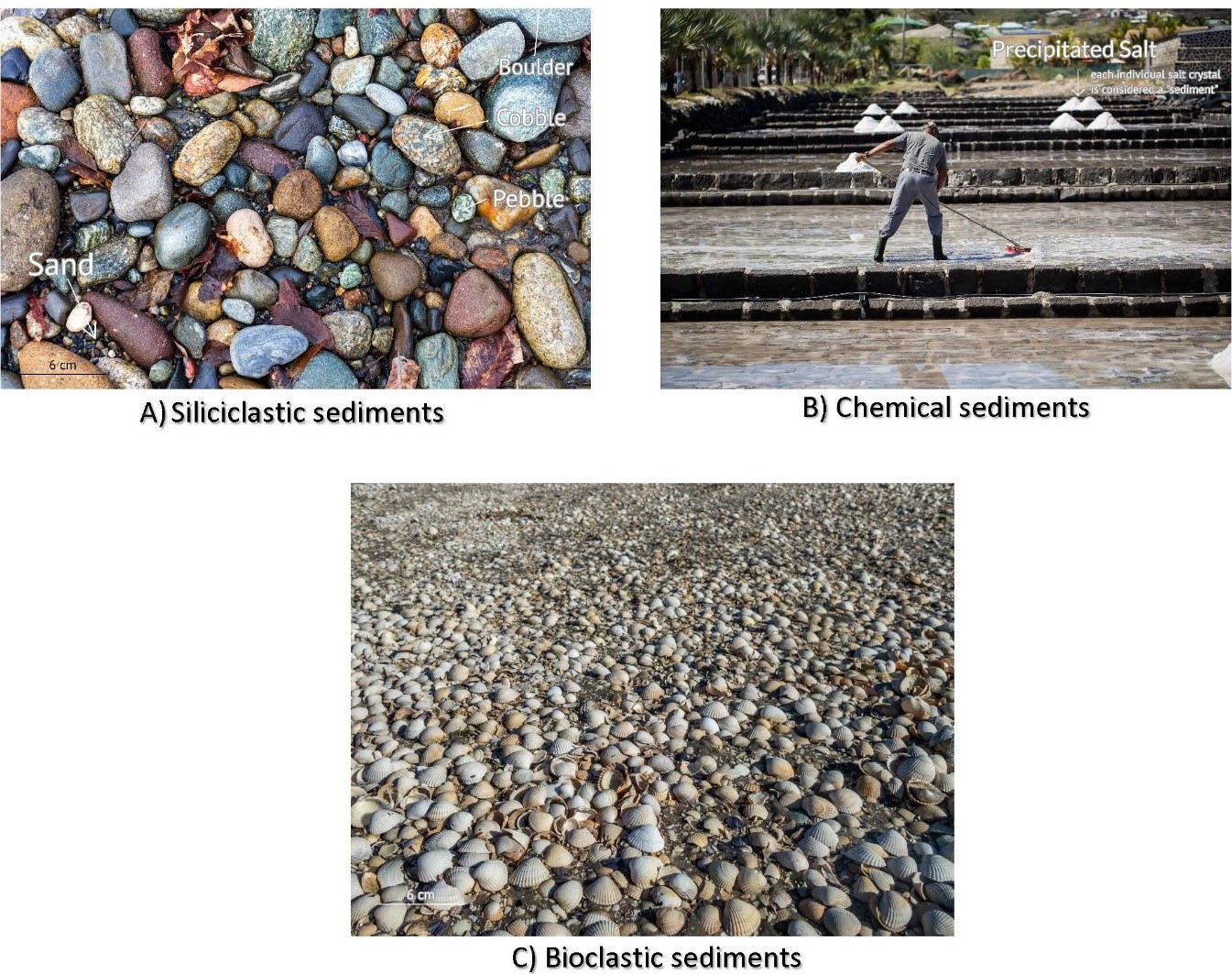
(a) Siliciclastic sediments derived from igneous rock fragments. (b) Chemical sediments of salt, precipitated from seawater in Mauritius, with each crystal forming a “sediment.” (c) Bioclastic sediments with each shell and shell fragment forming the “sediment.”
Transportation
Transportation can occur immediately after erosion, usually by the same eroding agent that carries the sediments downhill or, in aeolian transport, in the direction of the wind. Typically, water flowing over Earth’s surface, wind, and ice are the main agents of sediment transport, which moves them from positions of higher potential energy (hills and mountains) to positions of lower potential energy (valleys, lakes, and oceans). The strength of the water current as well as the distance of transport will leave an imprint on the sediments’ size, shape, and sorting (Table 4.1).
- Size If you have been fortunate enough to be able to hike to the headwaters of a major stream, you may have noticed that the sediments were very large (of the order of meters). This is because the transport distance for these sediments was short. They are located close to the rock outcrop from which they originated. Toward the middle and the mouth of the stream, the sediments get progressively smaller because they hit against each other and the sides and bottom of the stream. This process of attrition causes the sediments to be abraded (worn down) into smaller and smaller pieces the longer it is transported. Therefore, sediment size is a good indication of distance of transport. However, it must be noted that the energy of the stream can cause an increase in attrition, yielding smaller particles without the need for long transport distances.
- Shape The shape of sediments also indicates duration of transport, with particles becoming smoother and more rounded the longer the sediments are transported. As the sediments move along, they undergo attrition, which eventually, over long transport distances, removes angular edges. Note that the energy of the stream can also influence rounding. Therefore, more angular particles generally indicate shorter transport distances and smoother, more rounded particles indicate long transport distances.
- Sorting As sediments move with water, the largest particles will settle out of suspension first and the smallest will settle last. Larger sediments will generally be located close to the headwaters at the beginning of the stream, with medium-sized particles located in the middle of the stream and the smallest particles toward the end, at the mouth of the stream. This effectively “sorts” the sediments based on size along the stream profile. Note that particle settlement also depends on the energy of the stream, because more turbulent flow can allow for the suspension and transport of larger particle sizes (see Video 4.1). Therefore, poorly sorted sediments can indicate short transport distance and/or turbulent flow, while well-sorted sediments can indicate the converse (long transport and/or lamellar flow). A typical stream profile will be steeper at the beginning and become more gently sloping toward the end, resulting in a more energetic flow in the headwaters and low-energy flow at the mouth (Video 4.2).
Table 4.1. Siliclastic sediments’ size, shape, and sorting
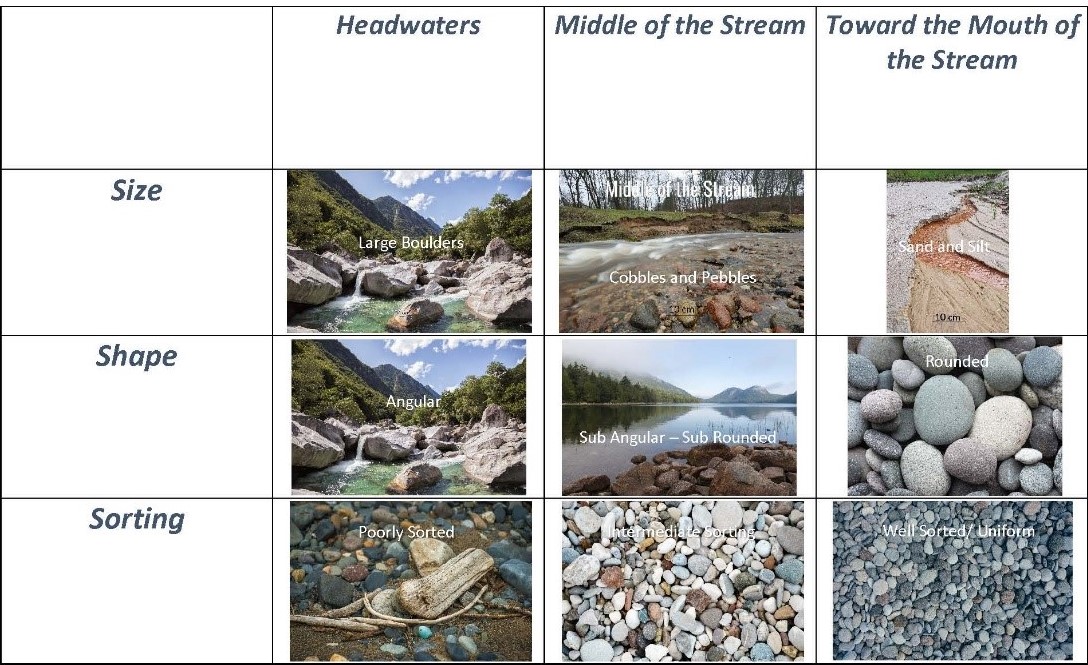
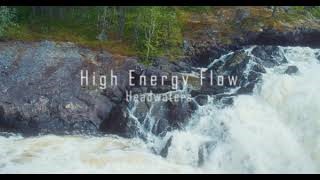
Deposition
Deposition occurs when sediments come to rest in environments of lower potential energy, such as basins, lakes, and oceans. Typically, in the absence of turbulent fluid flow, the largest sediments settle first and the smallest ones settle last. The way sediments are deposited will indicate the type of depositional process and environment. This is inferred by observing the sedimentary structures.
Essentially, there are three types of depositional sedimentary environments (see Interactive Figure 4.2):
- Continental These include sedimentary basins that exist on “land” in fluvial environments, lacustrine (lake) environments, deserts, and glacial environments.
- Shoreline These include sedimentary basins that exist at the margin of “land” and “sea” in the form of river deltas, beaches, and tidal flats.
- Marine These are sedimentary basins that exist in oceans and include the continental shelf, reefs, continental slopes, and deep-sea environments.
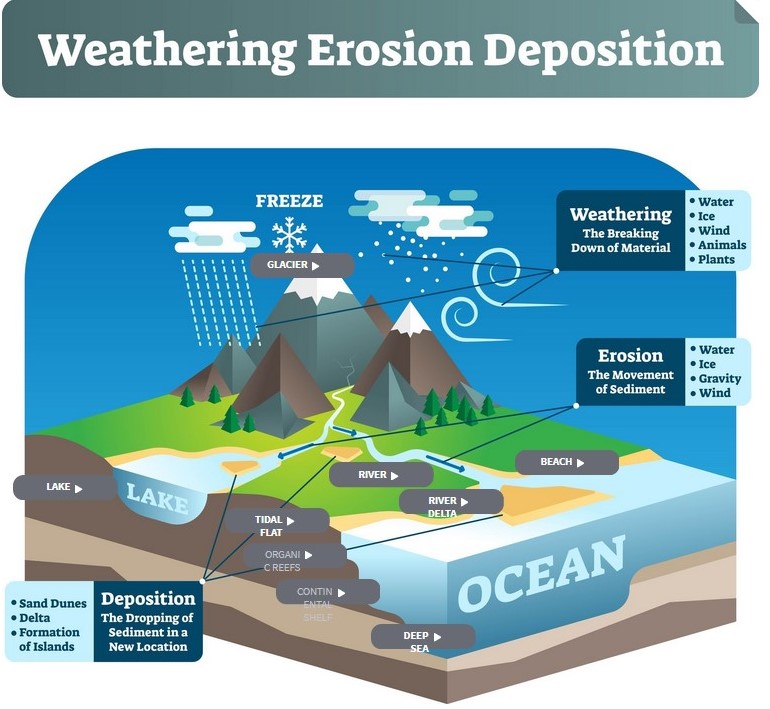
Click into each environment to see sediment type and transport agent. Click on the image to load the figure.
Furthermore, understanding the depositional environments and the associated sediments can offer insight into local and even global processes that occurred over deep geologic time. For example, if we observe an outcrop with sedimentary rock at the base made of sediments deposited in a deep marine environment followed by sediments deposited in a reef environment followed by siliciclastic sediments at the top, then this indicates sea-level fall. This type of an outcrop is called a bedding sequence.
Burial and Diagenesis
Burial occurs when previously deposited sediments are covered by subsequent sediment layers. Eventually the weight of the overlying layers compacts the underlying sediments, starting the process of diagenesis, which is the physical and chemical changes that sediments undergo leading to lithification.
The burial of sediments leads to their compaction, which squeezes water out. This leads to lithification, which turns the sediment into rock. However, compaction is not the only means by which sediments are lithified.
New minerals can also be introduced between the sediments by addition (that is, they can be deposited with the sediments) or precipitation. When this occurs, these minerals act like a cement that binds the sediments together into rock thus lithifying them, in a process called cementation.
Lithification of sediments can occur by compaction, cementation or a combination of the two (Figure 4.7).
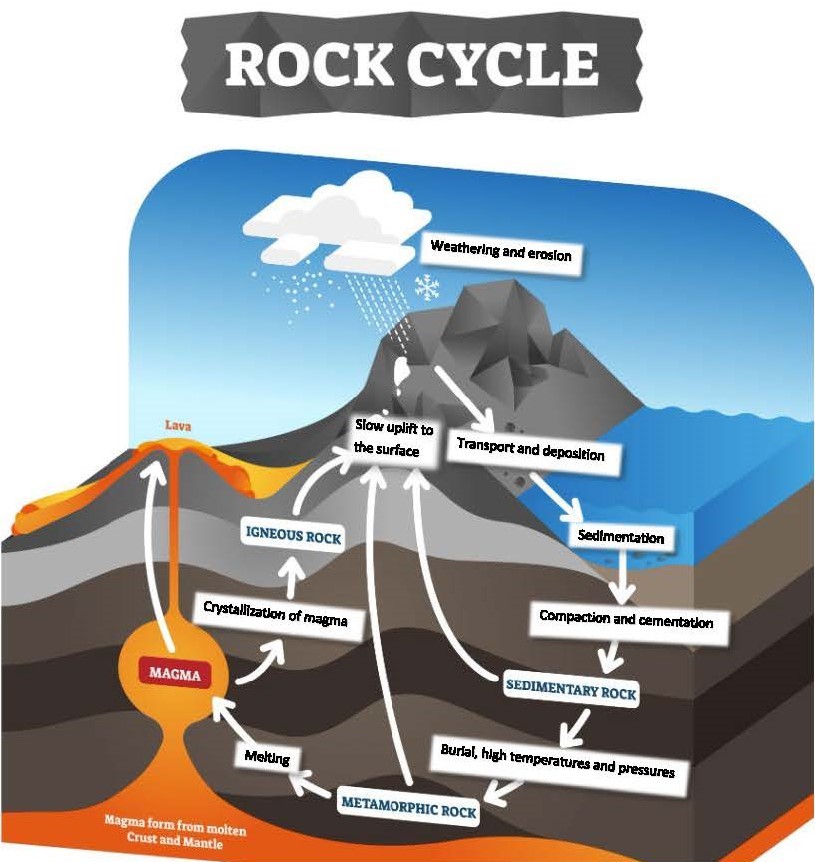
Sedimentary Rock Classification
Different types of sediment will result in different sedimentary rocks. Because sediments are classified as either siliciclastic, biological, or chemical, sedimentary rocks are classified under the same. Furthermore, the size of the sediments also influences the classification.
Siliciclastic sedimentary rock is derived from sediments that originate from existing igneous rock, typically from rock exposed on continental landmasses. Based on the size, these rocks are classified as claystone if the sediments are smaller than 0.0039 mm in diameter and as conglomerate if the sediments are larger than 2 mm in diameter (Interactive Figure 4.3).
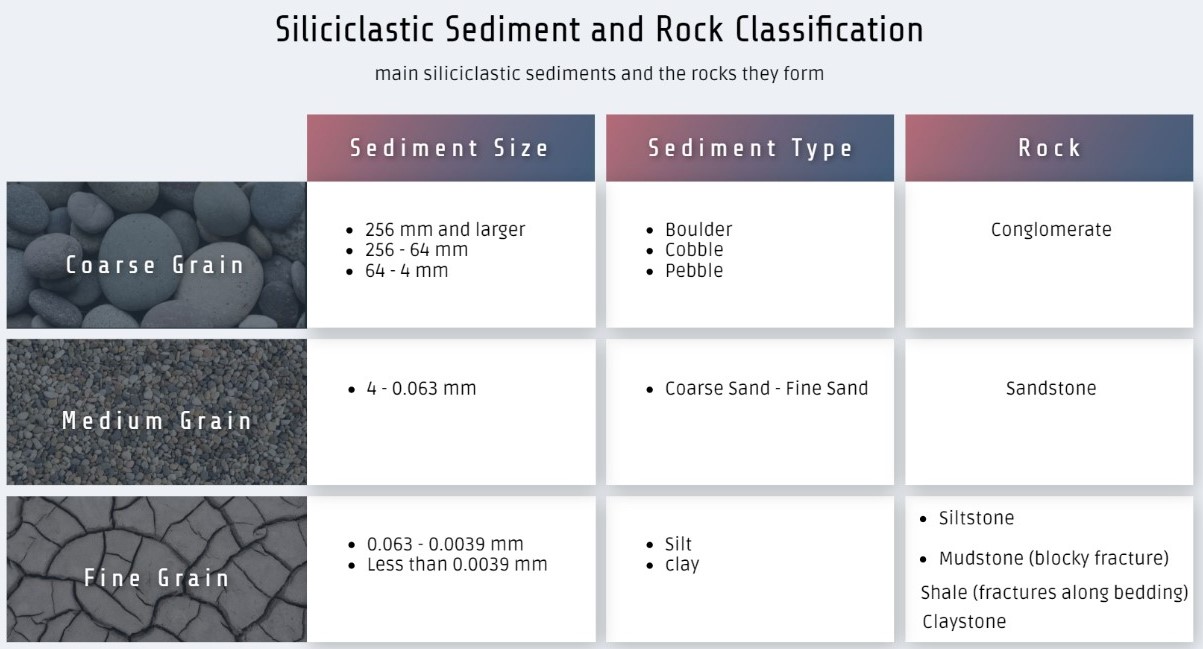
Click on each classification to see a photographic example.
Biological sedimentary rock (Interactive Figure 4.4) is derived from the shells and skeletons of dead organisms. These can be calcium carbonates and siliceous sediments from marine animals or hydrocarbons from organic matter. The calcium carbonate sediments form limestones, the siliceous sediments form chert, and the hydrocarbons form coal or anthracite.
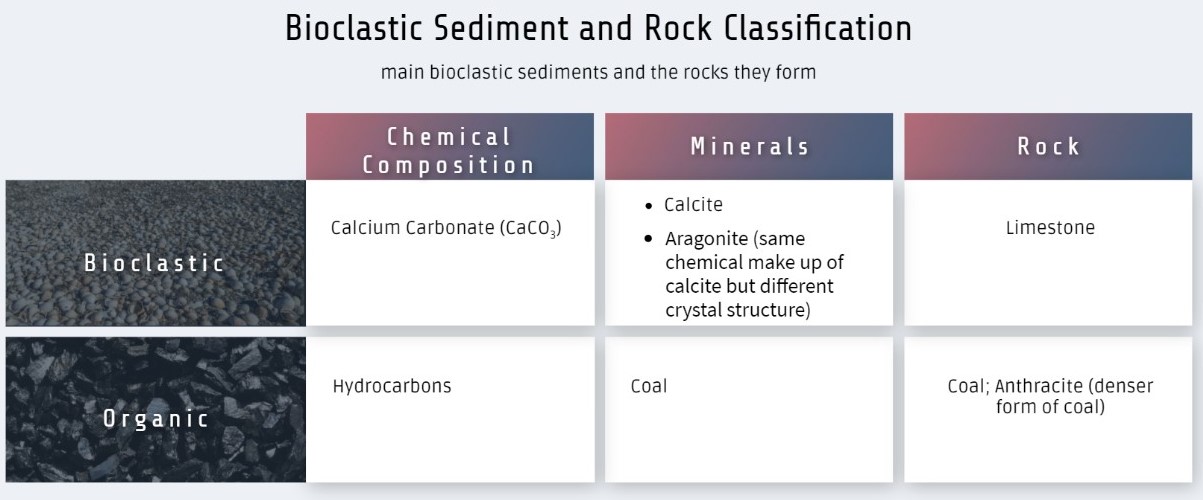
Click on each classification name to see a photographic example.
Chemical sedimentary rock is formed from the precipitation of minerals out of evaporating water (Interactive Figure 4.5), and the rocks they form are thus classified as evaporites.
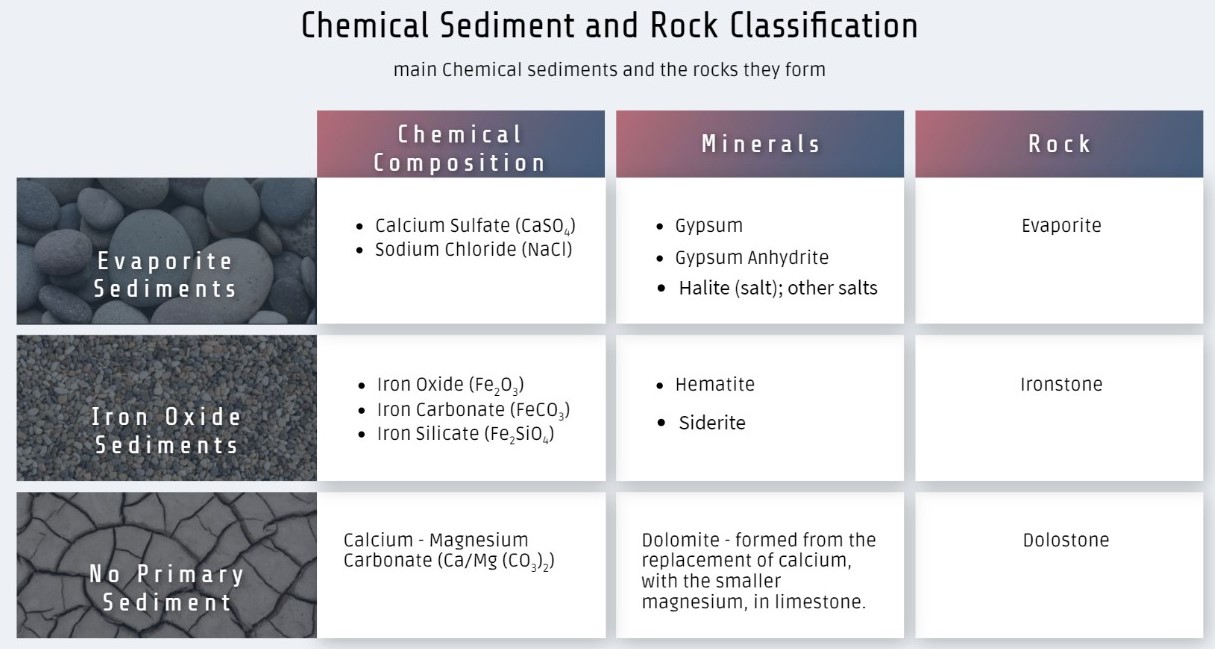
Click on each classification name to see a photographic example
4.5 Sedimentary Structures
As sediments are transported and deposited, the forces associated with these processes leave an “imprint” that is locked into the rock by diagenesis. When we observe how and where present-day sedimentary structures form, we can use the principle of uniformitarianism to infer that similar structures in rock must have formed in the same way. This allows us to determine the sediment transport processes and depositional environments of sedimentary rock.
Several structural features gets locked in the sedimentary rock at the time of deposition. These include stratification, graded bedding, ripples, dunes, cross-bedding, and bioturbation.
Stratification
Stratification (or bedding) is the layering that can be observed in sedimentary rock and marks the intermittent periods of deposition with the oldest sedimentary layers at the base and the youngest ones at the top. We can infer this because it is not possible for sedimentary layers to be deposited and lithified in mid-air while subsequent layers are deposited under it. This is called the principle of superposition.
Graded Bedding
Different layers of sedimentary rock are assumed to have been originally laid down in horizontal layers according to the principle of original horizontality. When these layers develop with significant differences in their sedimentary structure, we can infer that the depositional environment has changed significantly. Such a sequence of layered sedimentary rock is called a stratigraphic or bedding sequence. A stratigraphic sequence can be used to infer the macroscopic environmental changes that would yield the changes in the processes that create sedimentary structures in the rock.
For example, a typical stratigraphic sequence associated with a fluvial environment is a graded bedding sequence (Video 4.3). At the base, a graded bedding sequence would have larger sedimentary particles and larger cross-bedding layers; toward the middle, it would have medium-sized sediments and cross-lamination (small cross-bedding caused by ripple migration); and at the top would be horizontal layers of smaller silts and clays (muds). This sequence could be attributed to a flood sequence or a meander (Video 4.3).
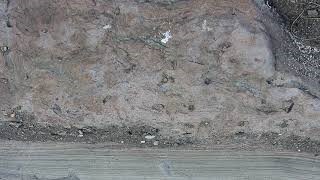
Click on the image to load the video.
Ripples and Dunes
Ripples and dunes are sedimentary structures formed by sediments transported at the base in flowing water (or wind). Ripples are of the order of centimetres in size while dunes are of the order of metres to kilometres in size. The more energetic the flow, the larger the structure, with low flow yielding ripples and high flow yielding dunes. Asymmetrical ripples and dunes indicate a unidirectional flow like that in rivers, while symmetrical morphology indicates intermittent flow in opposite directions, like in littoral zones (beaches where waves go in and out).
As the water (or wind) flows, ripples and dunes seem to migrate toward the direction of flow, creating an asymmetrical profile. Ripples and dunes developed due to unidirectional flow will have a convex profile facing upstream, called the windward slope or stoss slope, and a concave profile facing downstream, called the leeward slope or lee slope. This migration is somewhat of an illusion: the sediments on the windward slope migrate and are deposited on the leeward slope. This creates angled lee-slope layers that are successively built up while the layers on the windward slope are successively eroded. The net effect is a wholesale migration of the ripple or dune. However, in beach environments where flow is in opposite directions, sediments move from one slope to the other in equal amounts, creating a symmetrical profile (Figure 4.8).
Cross-Bedding
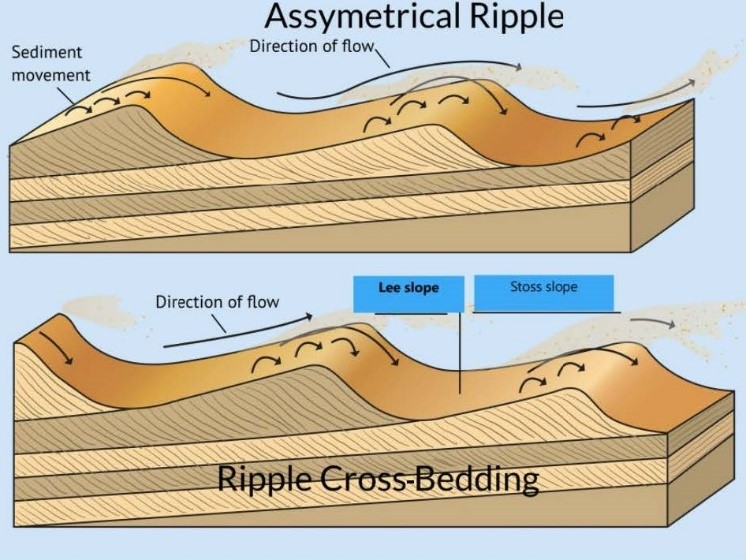
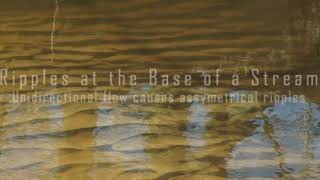
As the ripples or dunes migrate, they will eventually cover the angled layered sediments of previous ones, creating a sedimentary structure called cross-bedding. Smaller ripple migration creates a type of cross-bedding called cross–lamination, while larger dune migration creates trough cross-bedding (Figure 4.9).
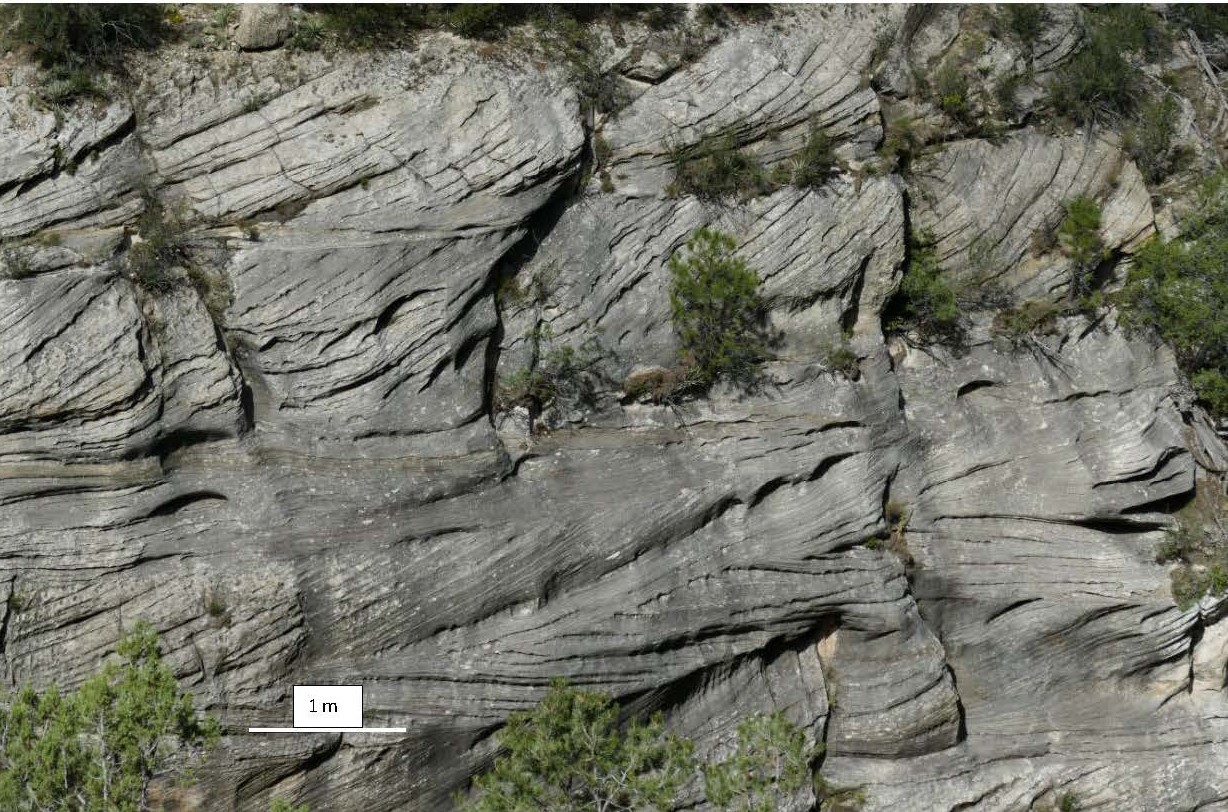
Bioturbation
After sediment deposition, benthic animals (that is, animals that live at the bottom of the sea or lake) that move through the substrate by burrowing will leave trails of their movement in the form of hollow tubes called bioturbation structures. Essentially, these tubes are fossils, and identifying the fossil can indicate the type of depositional environment (Video 4.5).
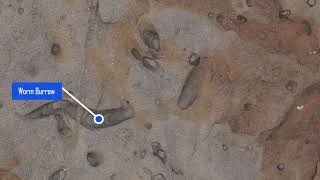
4.6 Metamorphic Rocks
Metamorphic rocks are born from existing rock that has had its chemical composition and/or its crystal structure transformed by changes in temperature and/or pressure. These changes occur in the solid form without any melting of the rock. Typically, minerals in rock begin to melt around 700 °C, however, the internal temperature of Earth does not rise to that until about 30 km deep. Metamorphism due to temperature starts at 250 °C (which is why placing hot pots on natural stone countertops is a big no-no). Because temperature rises 20–60 °C with every kilometre of depth, metamorphism due to Earth’s internal heat does not occur until about 6 km deep. Furthermore, metamorphism due to pressure starts at about 300 megapascals (MPa), or 3000 atmospheres, and can occur at any depth, as tectonic forces are capable of generating such pressures.
4.7 Processes in Metamorphic Rock Formation
Rock subjected to newly prevailing higher temperatures and/or pressures will reorganize its crystal structure to become more “comfortable” under these conditions. Pressure and temperature increase with depth in Earth’s crust, which causes the rocks to metamorphose. Therefore, metamorphic rocks can be sorted into grades, with low-grade metamorphic rocks occurring in low-temperature, low-pressure environments (250 °C; 300 MPa); intermediate metamorphic rocks occurring in moderate-temperature, moderate-pressure environments (500 °C; 500 MPa); and high-grade metamorphic rocks occurring in high-temperature, high-pressure environments (900 °C; 700 MPa; Interactive Figure 4.6).
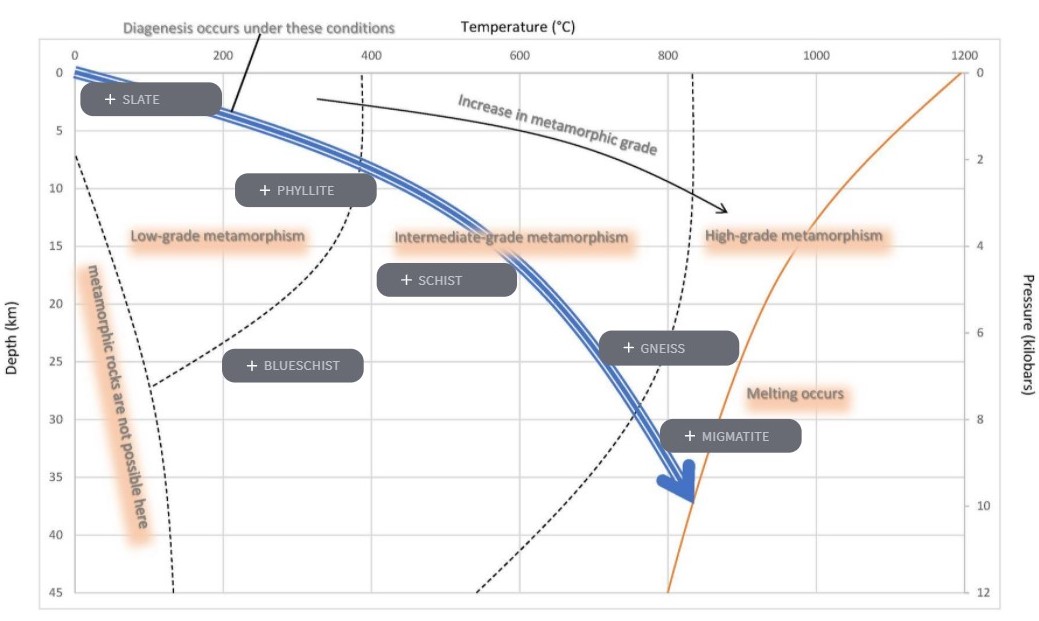
There are several ways in which new temperature/ pressure regimes can become incident on existing rock representing the different types of metamorphism as follows (see also Figure 4.10):
- Regional metamorphism Metamorphic rocks formed through regional metamorphism occur exclusively in association with convergent plate boundaries over areas that cover tens of several million hectares. This is a high-pressure environment due to the intense compressive forces generated by convergence, usually resulting in orogeny. However, temperature is low to intermediate, yielding low-grade to intermediate metamorphic rocks. Observation of these rocks indicates the presence of a convergent boundary even if that plate collision is no longer happening, because the rocks form the roots of mountain ranges associated with convergence.
- Contact metamorphism Contact metamorphism occurs in rock immediately adjacent to ascending magma in Earth’s crust. The incident heat radiating from the magma creates zones of metamorphism around it — in the intruded solid rock — that increase in intensity closer to the magma. Also, the magma can release pore fluids that can accelerate the change in mineral composition in the surrounding rock, thus accelerating metamorphism.
- Burial metamorphism This is usually the preliminary stage of metamorphism to occur in sedimentary rock after its lithification through diagenesis. Burial metamorphism occurs in low-temperature, high-pressure environments, yielding low-grade metamorphic rocks. The incident pressure required to start the metamorphic process is supplied primarily by the overlying sediments (or rock), which need to be very thick to generate pressures exceeding the 300 MPa required for metamorphism. Therefore, burial metamorphism occurs only in deep basins where sediments are thick enough to trigger metamorphism. These include areas along convergence zones from the continental shelf to the deep-sea trench (forearc). Temperature and pressure will continue to increase on these newly created metamorphic rocks as more sediments are added or as convergence proceeds. Eventually, these rocks will grade into intermediate metamorphic rocks associated with regional metamorphism.
- Metasomatism Metasomatism is caused by fluid exchange between the rock and any magmatic intrusions. Magma has water and carbon dioxide associated with it and can change the composition of the surrounding rock into which it intrudes. Also, water in the rock can be heated and flow through rock along fractures, removing minerals from or adding new minerals to the surrounding rock.
- Shock metamorphism Shock metamorphism occurs in response to the impact of a meteorite. Upon impact, kinetic energy is transformed into heat and pressure, which causes the rock in and around the impact crater to be metamorphosed. The effects of the elevated heat and pressure are short lived and are therefore localized.
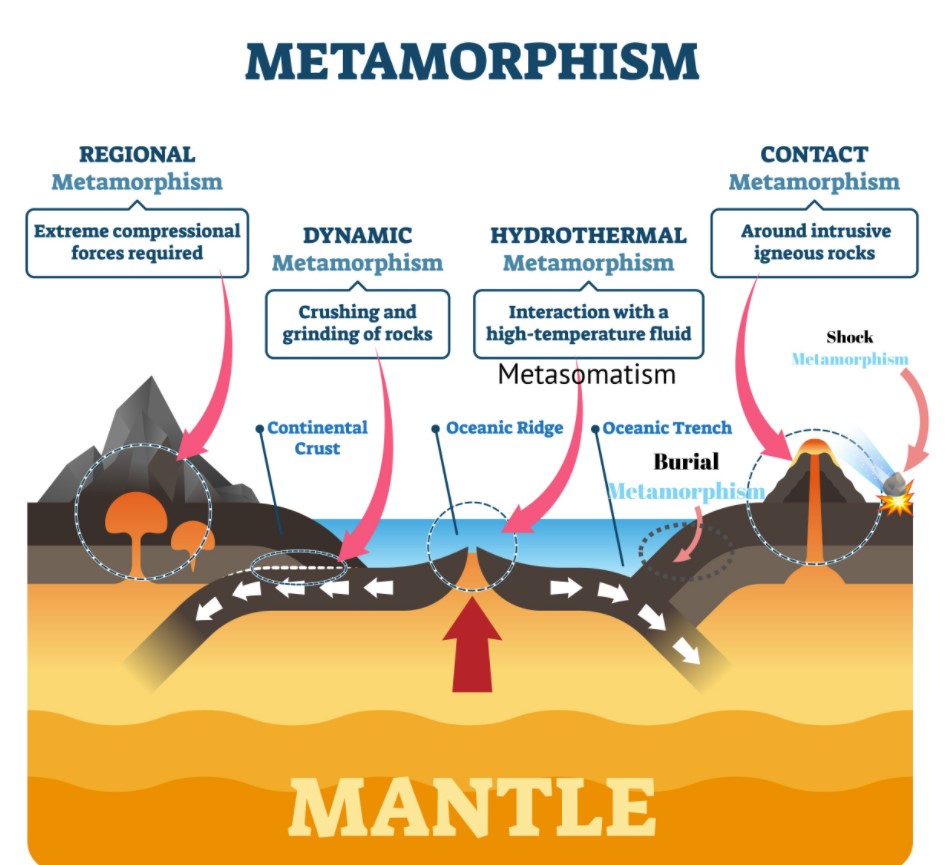
4.8 Metamorphic Textures and Metamorphic Rock Classification
On a macroscopic level, the response of the rocks to heat and pressure does not usually change the chemical composition of the rock (except in the case of metasomatism). This is why it is possible to determine the parent rock from which the new metamorphic rocks are formed.
Foliated Metamorphic Rocks
The pressure incident on existing rock is due to tectonic forces associated with convergence, yielding compressive forces. Compressive forces in rock affect the minerals and their crystal structure on a microscopic level. Platy minerals in the rock will tend to realign their long axes in an orientation perpendicular to the direction of the force. This is called foliation, and the stronger the force, the greater the alignment and thus the greater the foliation. This preferential alignment of platy minerals leads to the development in the rock of cleavage planes, which can be completely different from any other structures, such as bedding, already associated with the rock. To put it another way, the microscopic realignment of minerals has a macroscopic expression as cleavage planes in the rock.
In low-grade metamorphism, the compressive forces are low and produce weak foliation called slaty cleavage, while intermediate metamorphism produces schistosity and high-grade metamorphism produces banding (Figure 4.11).
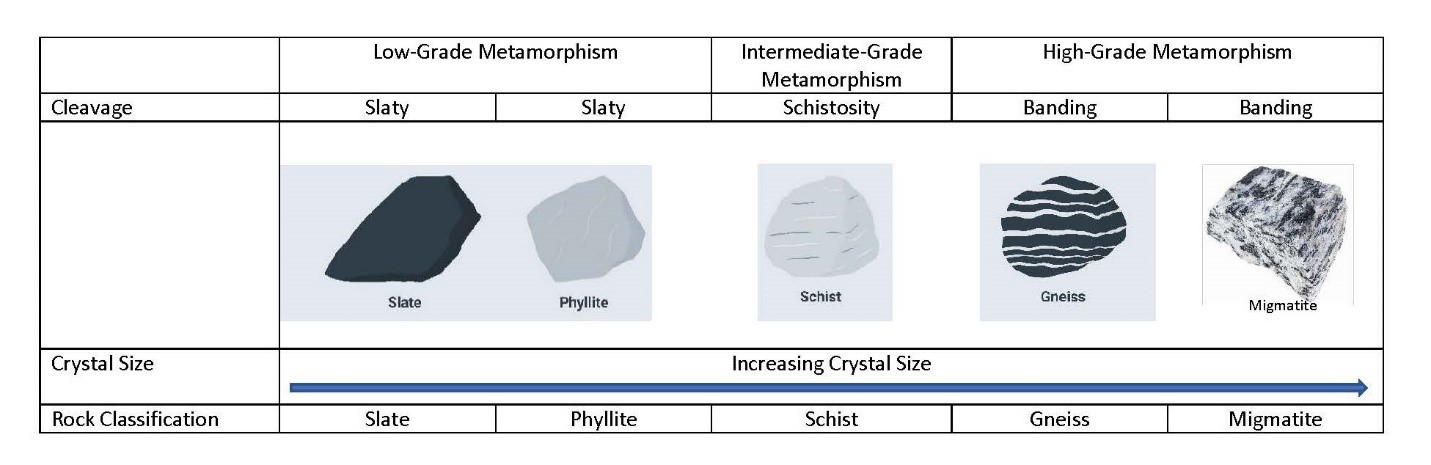
Furthermore, as the intensity of the metamorphism increases, so too do the crystal sizes of the minerals, which yields a coarse-grained texture. Because the type of foliation is tied to the metamorphic environment, it forms the basis for classification of foliated metamorphic rocks as follows:
Table 4.2 Classification of foliated metamorphic rocks

Non-foliated Metamorphic Rocks
In response to primarily being heated, existing rocks will develop non-foliated textures called granoblastic textures. This type of texture consists of fine to coarse grains of interlocking crystals that have little or no preferential orientation (foliation). Therefore, the classification of these rocks is based on the parent rock from which they originated as follows:
Table 4.3 Classification of granoblastic metamorphic rocks and their correlation to their parent rock and metamorphic environment. Click on the rock classification to see photographic examples.
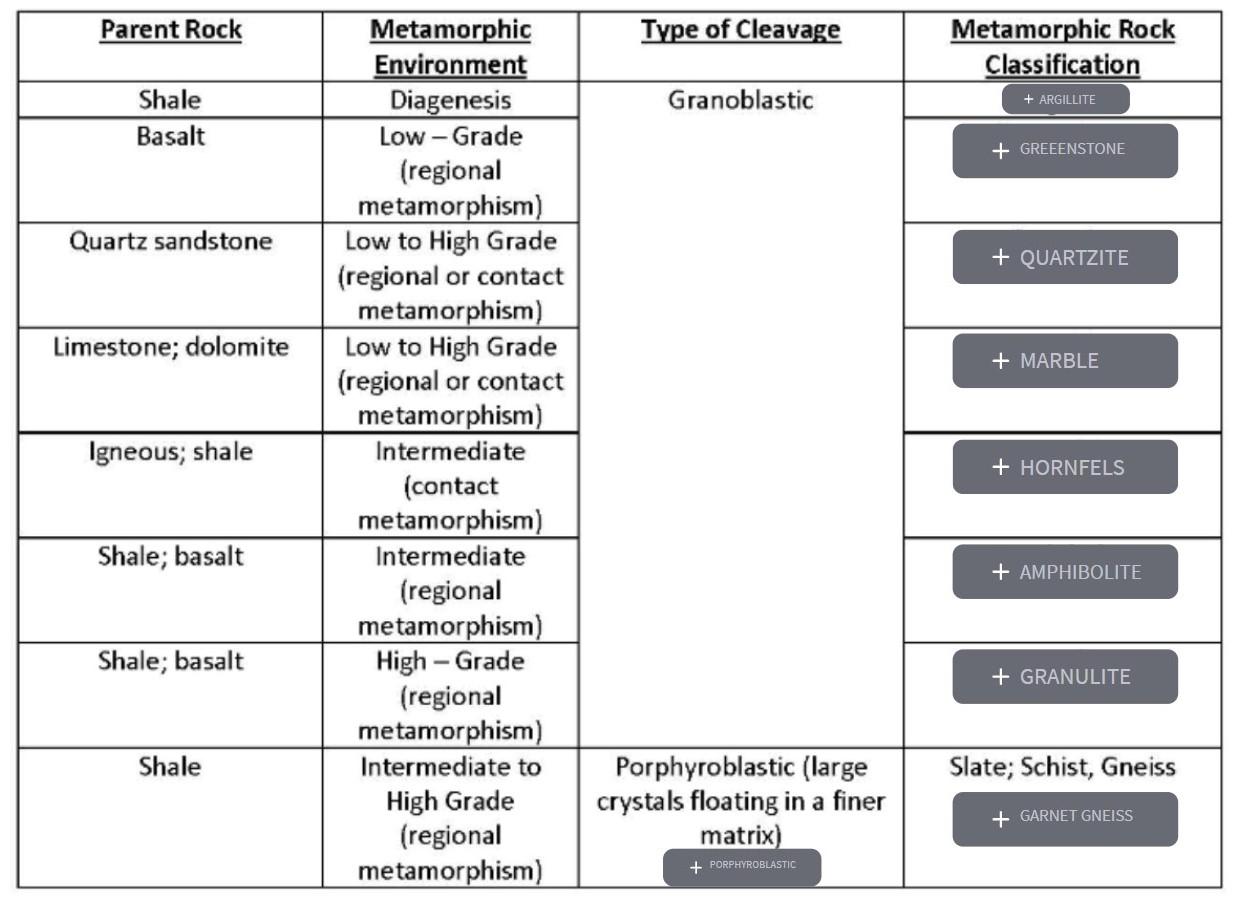
Metamorphic Facies
In response to specific combinations of temperature and pressure, certain predictable types of metamorphic rocks will develop. These are called metamorphic facies and can be correlated to types of metamorphism/metamorphic environments (Table 4.3 and Figure 4.12).
Table 4.4 Metamorphic facies and their corresponding metamorphic environment

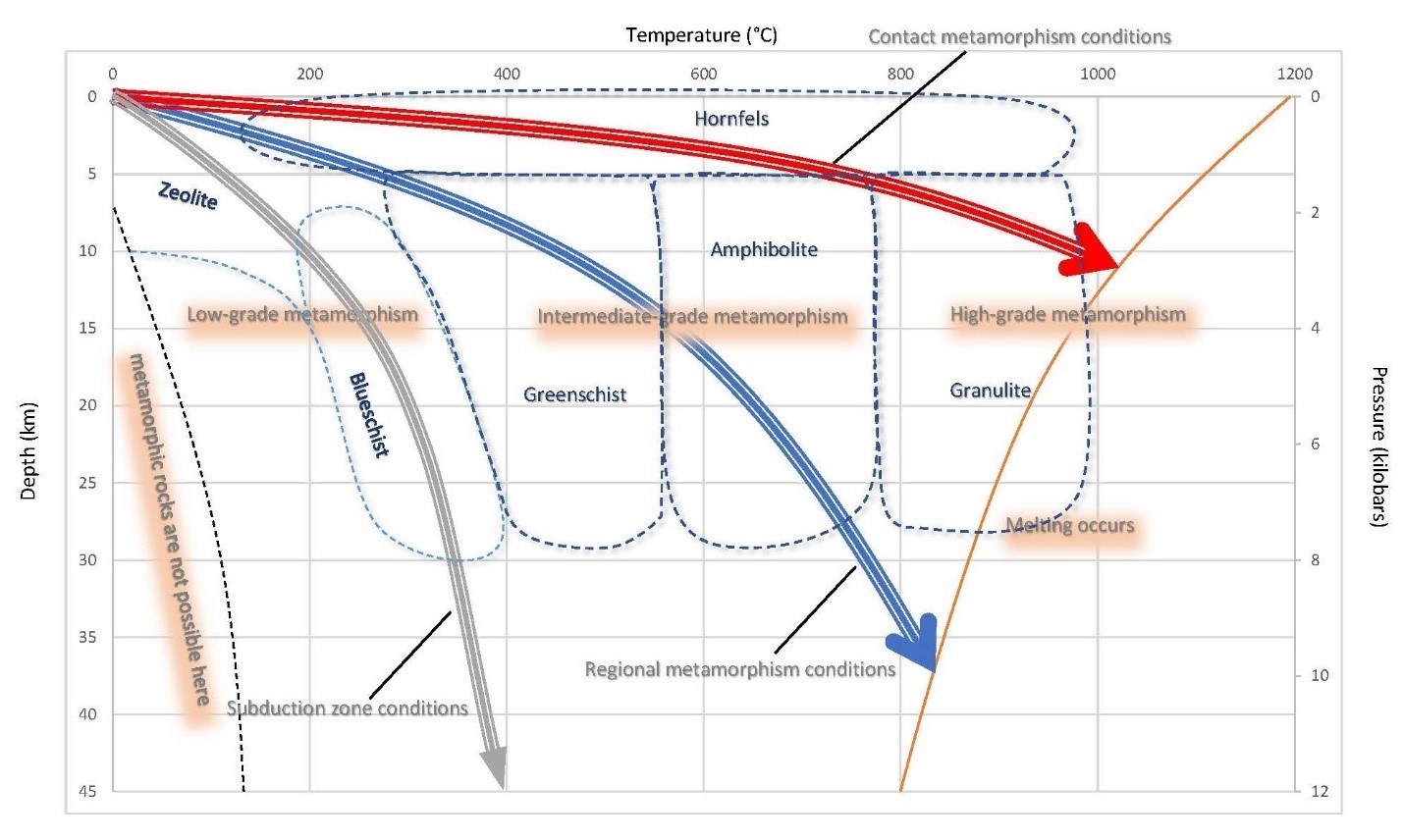
4.9 The Rock Cycle
If Earth were a planet with only high peaks and oceanless deep valleys, this would indicate that rocks were continuously created without being destroyed. Conversely, if no new rocks were being created, then Earth would be a waterworld with no landmass. Because neither of these scenarios describes Earth, then this means that rocks created at spreading centres and from volcanic eruptions must be destroyed elsewhere as a part of a cycle of conservation of Earth’s crust. This cycle is called the rock cycle; in it, created rock is broken down at Earth’s surface, through interactions with the hydrosphere, biosphere, and atmosphere, and at convergence zones (Figure 4.13).
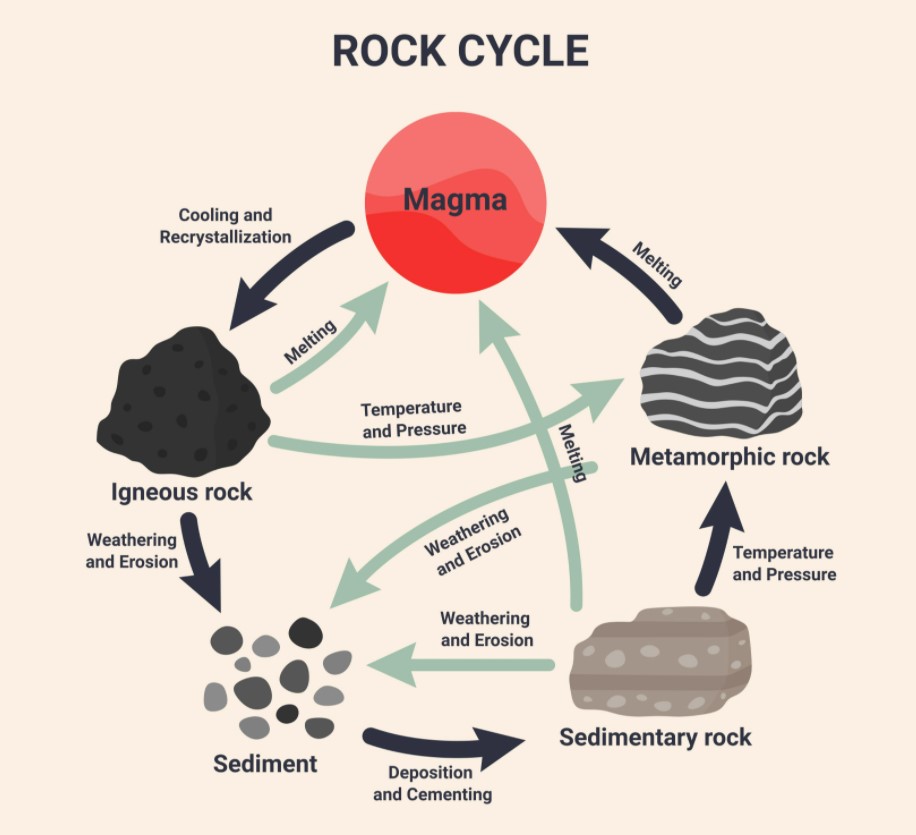
The term cycle tends to suggest a linear progression in changes, from the creation to destruction. However, in reality, the rock cycle is more complex than that. It is a cycle that shows how the different categories of rock relate to each other and through the processes that that form them and cause them to change into each other. Let us look at interrelations between the rocks and these processes (Table 4.4; see also Figure 4.13 above).
Table 4.5 Interrelations between rocks and processes in the rock cycle.

Naturally occurring aggregate of minerals
A visual description of the size of the minerals in the rock
The relative proportions of the different types of minerals in a rock
Rocks formed by the crystallization or solidification of magma or lava
Rocks formed from sediments
Rocks formed from existing rocks in Earth’s crust
Those that form from magma that cools and solidifies within Earth’s crust
The unmelted rock into which magma is intruded
A course-grained texture with different mineral sizes in intrusive igneous rock
In porphyritic igneous rock, a relatively larger mineral surrounded by relatively smaller minerals
In porphyritic igneous rock, minerals surrounding phenocrysts
Those that form from magma that erupts at the surface as lava
Rock solidified from airborne lava
The largest particles of pyroclastic igneous rock
The smallest particles of pyroclastic igneous rock
Rocks rich in feldspars and quartz minerals
Rock rich in metallic cations of magnesium and iron
Of igneous rock, that are rich in feldspars and quartz
Of igneous rock, with equal amounts of felsic and mafic minerals
Of igneous rock, that are rich in magnesium and iron cations
Of igneous rock, consisting of very little feldspars, no quartz, and considerable magnesium and iron
Extrusive igneous rocks
Intrusive igneous rocks
Formations of intrusive igneous rock
Magmatic intrusions that do not follow the prevailing geology of the country rock
The largest size of discordant intrusion
The smallest size of discordant intrusion
Magmatic intrusions that follow cracks and natural zones of weakness in the surrounding country rock
A concordant intrusion created when magma solidifies in a crack that cuts across the layers in the host rock
A concordant intrusion created when magma solidifies in cracks that are irregular and localized
A concordant intrusion created when magma solidifies along bedding directions
Smaller pieces of rock created by the weathering of existing rock
The process that breaks down rock at the surface of Earth
The process that removes newly created sediments
Of sediments, consisting mainly of silicates of igneous origin
Movement of sediments, especially by wind, water, or ice
The smoothing of rock particles
The following of irregular and overlapping paths by fluid particles
The following of smooth and generally parallel paths by fluid particles
The coming to rest of sediments in their environments
An outcrop consisting of multiple layers of sediments deposited in different environments
The covering of sediments by later sediment layers
The physical and chemical changes in sediment resulting in lithification
The creation of rock of through removal of water by pressure
The binding together of sediments by minerals to form rock
Rock derived from weathered igneous rock
Rock derived from shell and skeleton fragments of biological organisms
Rock derived from mineral precipitate
Layering in sedimentary rock
The order of layering in sedimentary rock
An order of layering of sedimentary rocked associated with a fluvial environment
Narrow structures formed by sediments transported at the base of flowing water or wind
Wide structures formed by sediments transported at the base of flowing water or wind
The convex, upstream-facing profile of ripples and dunes
The concave, downstream-facing profile of ripples and dunes
A sedimentary structure created by migrating ripples or dunes
The creating of hollow tubes in substrate by burrowing benthic animals
The transformation of rock over a large area in the crust, usually under mountain belts
Transformation of rock by the heat from adjacent magma
Transformation of rock by pressure from rock layers deposited above
Transformation of rock by the exchange of superheated water between the rock and magmatic intrusions
Transformation of rock by meteorite impact
The realignment of platy minerals such that their long axes is perpendicular to the direction of compressive force
Weak foliation produced by low compressive forces
In rock, coarse layering of different mineral compositions
In rock, a thick striped pattern of different mineral compositions
Fine to coarse grains of interlocking crystals with little or no foliation
Categories of rock that correspond to particular environments during which they were transformed into their current state
The creation and breaking down of rock in Earth’s crust and their interrelation